The Special Senses - Workings: how the special senses function
An individual's total experience of the external world—what he or she "feels"—is a blending of all the senses. The senses take in information about the world in the form of light waves, sound waves, and dissolved chemicals.

They convert those various forms into nerve impulses that are sent to the brain, which then interprets the impulses and gives them meaning in such a way that an individual sees, hears, smells, and tastes.
Sight
Light is a form of electromagnetic radiation—a form of energy carried by waves. The term electromagnetic radiation refers to a vast range of energy waves, including gamma rays, X rays, ultraviolet rays, visible light, infrared radiation, microwaves, radar, and radio waves. Of all these forms, only one can be detected by the human eye: visible light.
The human eye detects two types of visible light: direct light and indirect light. Direct light is light coming directly from a source such as the Sun or a lightbulb. Indirect light is light that has bounced off an object. People see trees, buildings, cars, and even the Moon through indirect light. Regardless of the type of light, an individual can see only if light rays are focused on the retina and the resulting nerve impulses are transmitted to the brain.
In short, sight occurs when light waves enter the eye by passing through the conjunctiva, cornea, aqueous humor, pupil, then the lens behind the iris. The lens focuses the light waves through the vitreous humor onto the retina. The rods and cones in the retina convert the light energy into electrical impulses. These impulses are then carried via the optic nerves to the brain, where they are interpreted as images.
When light passes from one substance to another of greater or lesser density, its waves or rays are refracted or bent. This is what happens when light waves pass from the surrounding air through the cornea and lens. The function of the lens is to focus the waves precisely on the retina. When the lens fails to do so, visual problems occur (see "Ailments" section below).
Researchers at Harvard University have found that everyone, not just fortune tellers, can see into the future. Tennis players routinely react to balls travelling over 100 miles (160 kilometers) per hour. The researchers conducted studies to determine how an individual could respond to an object that was moving faster than the eye had time to transmit its image to the brain.
The researchers found that the retina of the eye contains cells, called ganglion cells, that can calculate the future position of a moving object. The cells send nerve messages to the brain about the position of an object thousandths of a second before it actually arrives in that place in space.
This finding contradicts the notion that the eye acts like a simple camera, recording an image placed directly before it.
The lens changes shape depending on whether an object being viewed is far or near. The light waves from distant objects, generally over 20 feet (6 meters) away, approach the eye as parallel rays. In order to focus those rays on the retina, the lens does not change its normal, relatively flat shape, and the muscles of the ciliary body are relaxed. On the other hand, light waves from close objects tend to scatter and diverge or spread out. In order to focus those rays on the retina, the lens must bulge or become more spherical. To achieve this, the muscles in the ciliary body contract and the body forms a smaller circle. The elastic lens recoils and bulges in the middle (becoming convex). This process of changing the shape of the lens to keep an image focused on the retina is called accommodation.
When light rays strike the retina, they stimulate chemical reactions in the rods and cones. Different wavelengths of visible light (which result in different colors) bring about different types of chemical reactions. The reactions generate electrical impulses that are transmitted from the rods and cones by neurons. These neurons come together to form the optic nerve, which passes out through the rear wall of the eyeball.
The optic nerves from the two eyes then converge and nerve fibers from the inside portion of each optic nerve cross over to the other side. The nerves then separate again and travel to the occipital lobes of the cerebral cortex, the outermost layer of the cerebrum (largest part of the brain). The visual area in the right occipital lobe (on the right side or right hemisphere of the brain) receives nerve fibers from the right side of each eye. The visual area in the left occipital lobe receives nerve fibers from the left side of each eye.
When an image is formed on the retina by the light-bending action of a lens, it is an upside-down likeness of the object the light rays bounced off of. The image is also smaller than the original object (the farther away an object, the smaller its image on the retina) and reversed left to right. When the brain receives impulses containing information about these small, upside-down, reversed images from the eyes, it corrects the images to produce normal vision. Scientists still do not know how the brain does this.
Another ability of the brain is to bring together the two separate images (one from each eye) to form a single image. Each eye forms its own flat, twodimensional (with height and width) image from a slightly different angle. The brain compares and combines the two images to create a single image that is three-dimensional (one that also has depth). This ability of the brain, producing normal vision using both eyes, is known as binocular vision.
Hearing
Sound is produced by a vibrating object or body. As an object vibrates or moves back and forth, it causes molecules in the surrounding air to be pushed together or stretched apart. This disturbance in the air radiates outward in the form of waves that can travel through air, water, and solids. When these waves enter the ear, the effect is perceived as sound.
Frequency is the number of back-and-forth motions (called oscillations) a wave makes in a unit of time. Frequency is usually measured in cycles (vibrations) per second, and the unit used to measure frequency is the hertz (abbreviation: Hz). For example, 1,000 Hz is equal to 1,000 cycles per second.
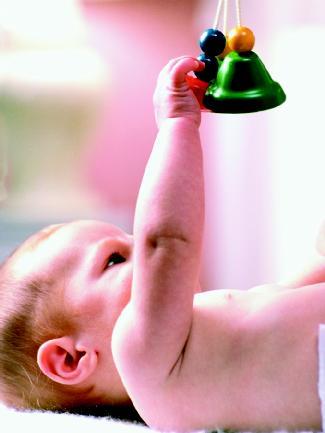
Objects can vibrate with a great range of frequencies, from only a few cycles (or vibrations) per second to millions of times per second. However, the human ear is able to detect only a limited range of those vibrations, generally those between 20 to 20,000 Hz. When the rate of vibration is less than 20 Hz, the sound is said to be infrasonic; when the rate is above 20,000 Hz, it is said to be ultrasonic.
When sound waves reach the outer ear, the pinna funnels the waves into the external auditory canal and toward the eardrum. As the waves strike the eardrum, it vibrates in response to the pressure or force of the sound waves. The initial vibration causes the eardrum to be pushed inward by an amount equal to the intensity of the sound. Once the eardrum is pushed inward, the pressure within the middle ear causes the eardrum to be pulled outward, setting up a back-and-forth motion.
The movement of the eardrum sets all three ossicles in motion. The vibrating pressure of the stirrup (the last ossicle) on the small opening leading to the inner ear sets the fluid in the cochlea in motion. The fluid motion causes a corresponding, but not equal, wavelike motion of the basilar membrane.
When the basilar membrane moves, it causes the small hairlike fibers on the top of the hair cells of the organ of Corti to bend. The bending of the hair cells causes chemical reactions within the cells themselves, creating electrical impulses in the nerve fibers attached to the bottom of the hair cells. The nerve impulses travel up the auditory nerves to auditory areas in the temporal lobes of the cerebral cortex of the brain, which interpret those nerve impulses as "sounds."
Sound waves usually reach the two ears at different times, allowing an individual to hear "in stereo." The brain uses this difference to determine from where in the surrounding environment the sound waves are coming. Scientists are not quite sure how the brain determines whether a sound is high-pitched or low-pitched, but they believe the sensation of pitch is dependent on which area of the basilar membrane vibrates (causing certain hair cells of the cochlea to bend). They also believe that the amount of vibration of the basilar membrane determines whether the brain will interpret a sound as loud or soft.
BALANCE. Besides hearing, the inner ear is also concerned with balance. The utricle and saccule (the membranous sacs in the vestibule of the inner ear) provide the brain with information about the body at rest. The tiny hair cells within these sacs project into a jellylike material that contains tiny mineral crystals. As the position of the head changes (while the body stays at rest), gravity causes the crystals to shift, pulling on the jellylike material. This movement bends the hair cells, and they are stimulated to generate nerve impulses that are sent to the cerebellum of the brain, informing it of the position of the head. The brain then uses this information to maintain equilibrium or balance.
Helping to maintain balance while the body moves through space are the fluid-filled semicircular canals. They provide the brain with information concerning rotational movements of the head. The three semicircular canals are arranged at right angles to each other, oriented in the three planes of space (up and down, right and left, forward and back). An area at the base of each semicircular canal contains sensory hair cells. Depending on the direction in which the body moves, the thick fluid in one or more of the semi-circular canals moves in the opposite direction and the hair cells are bent. This stimulates the hair cells to generate nerve impulses that are sent to the cerebellum. The brain then uses the information to maintain balance (by coordinating muscle movements) while the body is moving.
Smell
Scientists believe there are only a few basic odors or scents that combine to form all other odors. However, they have found it hard to agree on the number of basic odors. Estimates range from seven to fifty or more. Although many animals have a sense of smell far superior to that of humans, the human nose is capable of detecting over 10,000 different odors, even some that occur in extremely minute amounts in the air.
For smelling to occur, chemicals in the air must enter the nostrils into the nasal cavity. There, they must be dissolved in the mucus covering the olfactory epithelium. Once dissolved, the chemicals bind to the olfactory hairs, stimulating the olfactory receptors to send nerve impulses along the olfactory nerves to the olfactory areas in the temporal lobes of the cerebral cortex. The brain interprets the impulses as a specific odor or odors.
Olfactory information is also sent to the limbic system, a horseshoe-shaped area of the brain concerned with emotional states and memory. Thus, the impressions formed by odors or smells are long-lasting and very much a part of an individual's memories and emotions.
Taste
Although there are only four general types of taste receptors—sweet, sour, salty, and bitter—people experience many different tastes because the chemicals in foods stimulate different combinations of the four receptors.
The mechanism for tasting is similar to that for smelling. Chemicals from foods and liquids that are dissolved in the saliva come in contact with the taste hairs of the gustatory cells. The cells are then stimulated to generate nerve impulses that are carried by three cranial nerves to the parietal and temporal lobes of the cerebral cortex. The brain then interprets those impulses as taste sensations.
Scientists have discovered that individual tasting abilities and preferences for specific foods are partially hereditary. Some people are genetically programmed to have more taste buds than others and, as a result, taste more flavors in a particular food. Additionally, culture and familiarity with foods greatly influence taste preferences. Foods that are a tradition in certain cultures may be unappealing to those who are unfamiliar with them. A taste for a particular food usually develops as a person consumes it more frequently.
The smell, texture, and temperature of foods also affect taste. People often first experience the flavor of a food by its odor. When an individual's sense of smell is decreased due to congestion from a cold or flu, that person frequently experiences a reduced ability to taste. Some people will not eat pears because of the fruit's gritty texture, while others would not think of drinking cold coffee.
Comment about this article, ask questions, or add new information about this topic: