The Lymphatic System - Workings: how the lymphatic system functions
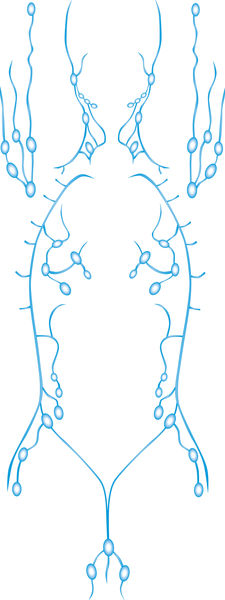
The exchange of materials (oxygen, carbon dioxide, nutrients, and wastes) between the blood and the cells in the body occurs through the capillaries. In the body of an average person, over the course of an average day, roughly 25.4 quarts (24 liters) of plasma fluid are forced out of the capillaries into the interstitial fluid surrounding the cells. After bathing the cells, providing them with nutrients, and picking up their wastes, this fluid is drawn back into the capillaries. However, only 85 percent of the total fluid is drawn back into the bloodstream. The remaining 15 percent, roughly 3.8 quarts (3.6 liters), remains in the interstitial fluid.
If this small amount of fluid were allowed to accumulate over even a brief period of time, massive edema (swelling caused by excessive bodily fluid) would result. If left unchecked, the body would blow up like a balloon, tissues would be destroyed, and death would take place. This condition is prevented by the presence of lymph capillaries, which run alongside blood vessels in most tissue spaces. The lymph capillaries act as "drains," collecting the excess fluid and returning it to the venous blood just before the blood reaches the heart.
Proteins and other large molecules dissolved in the interstitial fluid cannot be absorbed by the blood capillaries. Because the walls of lymph capillaries are much more permeable (allow material to pass through easily), these large substances enter the lymph capillaries and are eventually returned to the blood.
This function of lymph capillaries is particularly important in the small intestine. Whereas carbohydrates and certain other nutrients are small enough to pass directly from the intestine into the bloodstream, fats are not. Lacteals (lymph capillaries in the small intestine) are able to absorb fats and other nutrients that are too large to enter blood capillaries. After digestion, the lymph in lacteals contains as much as 1 to 2 percent fat. Milky-white in appearance, this thick mixture of lymph and tiny fat globules is called chyle. It becomes mixed with the blood after lymph drains into the thoracic duct.
After the vessels of the lymphatic system have collected excess fluid, cellular wastes, proteins, fats, and other substances too large to enter the blood capillaries directly, they return all this material to the bloodstream. Before advancing to the heart and reentering the circulatory system, however, any fluid and matter that enters the lymphatic system must pass through at least one lymph node. It is very likely that foreign substances, such as viruses, bacteria, and even cancer cells, are a part of the lymph that has been collected from all parts of the body. Even the dark, gritty debris of polluted city air finds its way from the lungs of city dwellers into the lymph. Without the filtering abilities of lymph nodes, these foreign substances would overrun the body.
Florence Rena Sabin, a pioneering medical researcher and educator, helped settle a longstanding controversy: how the lymphatic system developed in the human body. In a field dominated by men, Sabin achieved much: she was the first woman faculty member at Johns Hopkins School of Medicine (as well as its first female professor) and the first woman elected to membership in the National Academy of Sciences.
Born in Central City, Colorado, in 1871, Sabin graduated from Smith College in Massachusetts with a bachelor of science degree in 1893. Three years later, she entered Johns Hopkins School of Medicine. After graduating in 1900, Sabin was accepted as an intern at Johns Hopkins Hospital, a rare occurrence for a woman at the time. But her interest lay in research and teaching, and she was soon awarded a fellowship in the department of anatomy at Johns Hopkins School of Medicine.
In this position, Sabin began her studies of the lymphatic system. At the time, researchers were split over whether the lymphatic system developed separately from the vessels of the cardiovascular system. To find the definitive answer, Sabin studied pig embryos because they developed in a manner similar to humans. (An embryo is an organism in its earliest stages of development.)
After painstaking research, Sabin discovered that lymphatic vessels did, in fact, arise from veins. She found that the outer layer of cells on veins sprouted buds, much like stems growing out of the branches of trees. As these stems grew outward, they connected with each other. This proved that the lymphatic system developed entirely from existing vessels in the body. For her work on lymphatics, Sabin won the 1903 prize of the Naples Table Association, an organization that maintained a research position for women at the Zoological Station in Naples, Italy.
Throughout her career, Sabin continued to study the lymphatic system and the body's immune responses. She paid special attention to the bacterium that causes tuberculosis, an infectious disease marked by lesions on the lungs, bones, and other body tissues.
Sabin died in Denver, Colorado, in 1953.
Immunity is the body's ability to defend itself against pathogens or other foreign material. The human body is awash in a sea of infectious agents such as bacteria, viruses, fungi, and parasites. It provides an ideal habitat for many of them. If these organisms were to break through the barriers erected by the body, they would ultimately destroy it. To protect itself, the human body relies on two lines of defense: nonspecific and specific.
The nonspecific defense system
The nonspecific defense system prevents the entry and spread of foreign microorganisms throughout the body. It is a general defense that does not discriminate between one threat and another; it responds to protect the body from all foreign substances, whatever they are. Present at birth, it includes physical barriers and cellular/chemical defenses.
PHYSICAL BARRIERS. Physical barriers are the body's first line of defense against disease-causing microorganisms. These barriers include the skin and mucous membranes. The skin, the outer tissue covering of the body, forms a strong physical barrier to most microorganisms that swarm on its surface. Microorganisms are unable to penetrate the upper layers of dead skin cells. In addition, the secretions from sweat and sebaceous (oil) glands in the skin contain chemicals that inhibit the growth of bacteria.
The membranes that line all body cavities open to the exterior—digestive, respiratory, urinary, and reproductive tracts—secrete or release sticky mucus that traps microorganisms. To further trap or destroy foreign invaders, the membranes in some of these tracts also contain hairs or secrete a variety of protective chemicals.
For instance, nasal hairs in nasal passages filter and trap large microorganisms before they enter the trachea or windpipe. Those invaders that do get by are often caught in the cilia, the microscopic hairlike structures projecting from the mucous membrane lining the trachea. The cilia wave back and forth in rhythmic movement, and trapped particles are swept along up through the trachea and into the throat. From here, the mucus and particles are either expelled by sneezing or coughing or swallowed into the stomach.
The mucous membrane of the stomach secretes hydrochloric acid and protein-digesting enzymes. Both kill microorganisms. Bacteria is also destroyed by saliva in the mouth and tears in the eyes, which each contain an enzyme that breaks down the walls of bacteria cells.
PHAGOCYTES. If a microorganism is able to break through the body's surface barriers, such as through a scrape or cut, the body uses an enormous number of cells and chemicals to protect itself. Any bacteria or foreign material that enters the body is immediately confronted by phagocytes. These are types of white blood cells that destroy foreign particles by surrounding them and engulfing them—a process called phagocytosis. The largest group of phagocytes are macrophages (literally, "big eaters"). A macrophage, which arises from a white blood cell called a monocyte, can "eat" up to 100 bacteria.
Another group of cells in the nonspecific body defense line are natural killer or NK cells. These type of lymphocytes are a unique group of defensive cells. They constantly patrol the body, floating in blood and lymph, seeking out antigens on foreign or abnormal body cells (such as those infected with viruses or cancer). NK cells can react against any such cells—they are not specific. Unlike macrophages, however, they are not phagocytic. When confronted with a foreign or abnormal cell, an NK cell secretes proteins that dissolve the target cell's membrane and the cell quickly disintegrates.
INFLAMMATION. Inflammation is the body's second line of defense. It develops whenever body tissues are damaged by physical injury or infected by bacteria and viruses. Inflammation is localized, meaning it occurs only where the injury or infection has taken place. The four major symptoms marking inflammation are redness, heat, swelling, and pain.
The inflammation process begins when damaged cells release chemicals such as histamine into the surrounding interstitial fluid. Histamine and the other chemicals cause the local blood vessels to expand, which increases blood flow to the area (accounting for the redness and heat). This, in turn, brings more oxygen, nutrients, and white blood cells into the area to fight the infection and begin the healing process. The heat produced by the additional blood increases the rate at which nearby cells "work" and creates unfavorable conditions for bacterial growth. As the blood vessels expand, they also become more permeable, allowing plasma from the bloodstream to seep into the interstitial spaces. This causes edema or swelling, which then activates local pain receptors. Both swelling and pain restrict movement in the area, further aiding healing.
FEVER. The body also uses heat in a more general way to fight infection: fever. A fever is a continued overall body temperature greater than 99°F (37°C). The hypothalamus is a portion of the brain that controls many body functions, including body temperature. In a sense, the hypothalamus is the body's thermostat. When white blood cells and macrophages are exposed to bacteria and other foreign invaders, they secrete chemicals that signal the hypothalamus to raise body temperature. The added heat in the body deters the growth of bacteria and speeds up the repair processes in the damaged cells. However, the temperature range at which fevers are beneficial is limited. High fevers—with temperatures over 104°F (40°C)—can damage many organ systems.
INTERFERONS. In 1957, scientists discovered another nonspecific defense mechanism: small protein compounds called interferons. Once infected with a virus, damaged cells help defend nearby uninfected cells by releasing interferons. These proteins travel to normal cells and bind to their membranes. Once attached, the interferons cause the normal cells to produce substances that prevent the virus from reproducing within those cells.
The specific defense system
The specific defense system is sometimes referred to as the body's third line of defense. Like the nonspecific defense system, it seeks out foreign invaders and acts to disable or destroy them. Unlike its sister system, however, the specific defense system recognizes and acts against particular foreign invaders or abnormal body cells. It is not limited in its actions to the initial site of infection, but functions throughout the entire body. It also has a "memory," mounting a quicker and stronger attack against viruses and bacteria when it encounters them a second time. For all these reasons, the specific defense system is much more effective in defending the body and establishing immunity.
The two separate but overlapping classes of lymphocytes—B cells and T cells—lead the specific immune response. The class called into play depends on the type of foreign or infected cells present in the body. B cells defend against invading bacteria and viruses. Because B cells produce antibodies to attack specific antigens, the immune response launched by B cells is called antibody-mediated immunity. T cells also defend the body against viruses, parasites, fungi, and other invaders. In addition, they attack body cells infected by viruses and bacteria and those that are cancerous. Because T cells attack pathogens directly, their immune response is called cell-mediated immunity.
T CELLS AND IMMUNITY. Cell-mediated immunity is triggered when a macrophage encounters a foreign or infected cell. The macrophage engulfs the cell, breaks it down, then "displays" fragments of that cell's antigens (chemical identification markers) on the outer surface of its membrane. The macrophage then "presents" itself to a T cell that been trained to recognize those specific antigens as nonself or foreign. Once this occurs, the T cell becomes activated and divides into four types: helper T cells, killer (or cytotoxic) T cells, suppressor T cells, and memory T cells. These T cells then travel to the point of infection in the body.
Helper T cells direct or manage the immune response, not only at the site of infection but throughout the body. They stimulate the production of more T cells and antibodies by B cells. They also attract other types of protective white blood cells into the infected area and spur the actions of killer T cells. As their name suggests, killer T cells are responsible for the destruction of foreign or abnormal cells. They kill a target cell by binding with it and injecting a chemical into the target cell, rupturing its membrane.
Once the invading or abnormal cells have been destroyed and the infection has been brought under control, suppressor T cells take over. They release chemicals that slow down and eventually stop the action of other T cells and B cells. This prevents the immune response from remaining active when it is not necessary and possibly harming normal body cells.
Most of the T cells gathered to fight infected or abnormal body cells die after only a few days. Those that remain are called memory T cells. Their job is to "remember" the antigens of each invader or abnormal cell so that if they appear again in the future, the body will be able to respond quickly and efficiently. Memory T cells are long-lived, often surviving in the body for up to twenty years or more.
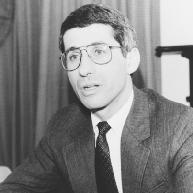
Since 1981, Anthony S. Fauci has play a significant part in AIDS research in the United States. As director of the National Institute of Allergy and Infectious Diseases (NIAID) and the Office of AIDS Research at the National Institutes of Health (NIH), Fauci supervises the ongoing investigation of how the disease works and the development of vaccines and drugs to treat and cure it.
Born in 1940 in Brooklyn, New York, Fauci attended Cornell University Medical School, graduating in 1966. Three years later, he became a clinical associate in the Laboratory of Clinical Investigation of NIAID. He has worked for the NIH ever since.
From the beginning of his medical career, Fauci has focused on the functioning of the body's immune responses and the impact of infectious diseases on them. By 1971, he had found cures for three diseases affecting body immunity.
When AIDS became recognized in the United States in 1981, Fauci was already deputy clinical director of NIAID. He immediately shifted the focus of the Laboratory of Clinical Investigation to the investigation of AIDS. The lab then made the important discovery of how HIV affects helper T cells.
During his tenure at the NIH, Fauci has worked not only against AIDS but against government indifference to the disease. He has won increasingly larger budgets for research. Fauci and his laboratory teams continue to search for an AIDS vaccine and to develop drug therapies to try to help those people already afflicted.
B CELLS AND IMMUNITY. Antibody-mediated immunity is activated when a B cell encounters its triggering antigen on the surface of a macrophage. B cells can also be activated by chemicals secreted by helper T cells that have already encountered specific antigens. In either case, once a B cell has been activated, it divides numerous times into plasma cells and memory B cells.
The many plasma cells that descend from a B cell have the ability to produce antibodies (also called gamma globulins). These Y-shaped proteins attach themselves to the foreign antigen. Each antibody produced matches only one antigen, much like a key matches a specific lock. Often the fit is precise; other times it is not. Regardless, once the antibody interlocks with the antigen, it either transforms the cell into a harmless substance or marks the entire antibody-plus-foreign cell package for destruction by phagocytes.
Memory B cells perform the same immunity function as memory T cells: to quicken and strengthen the immune response. Long-lived, they remain in the body to face the same antigens should they appear again. Once that exposure takes place, the memory B cells immediately divide and multiply into antibody-secreting plasma cells.
TYPES OF BODY IMMUNITY. The human body is protected by two types of immunity: genetic and acquired.
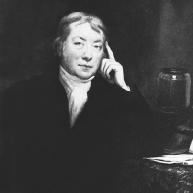
The first effective vaccine was developed against smallpox, a fast-spreading disease characterized by high fever and sores on the skin. Prior to the eighteenth century, the disease was common and often fatal. In 1796, while a smallpox epidemic raged in Europe, English physician Edward Jenner (1749–1823) tested a theory. He had observed that people who had been in contact with cows did not develop smallpox. Instead, they developed cowpox, a similar, milder disease of cows that was not a threat to human life. Jenner believed that individuals who had been infected with cowpox had developed an immunity to the more severe human smallpox.
Taking cowpox fluid from the sores of a milkmaid named Sarah Nelmes, Jenner rubbed it into cuts on the arm of eight-year-old James Phipps. A few days later, the boy came down with a mild case of cowpox, but soon recovered. Six weeks later, Jenner gave young Phipps some fluid from a person who had smallpox and he was not affected. His body had developed an immunity against the disease. Jenner called his procedure vaccination, from the Latin vaccinus , meaning "of cows."
In 1980 the World Health Organization declared the eradication of smallpox, the only infectious disease to be completely eliminated.
Genetic or inherited immunity is present in the body from birth. It does not involve an immune response or the production of antibodies. Because of the structure of human DNA (deoxyribonucleic acid; the genetic material determining the makeup of all cells), humans are not subject to certain diseases that dogs and other animals are, and vice-versa. For example, humans cannot contract distemper; however, dogs and cats can. Conversely, humans can suffer from measles; dogs and cats cannot. The genetic makeup of human cells (and of animal and plant cells, also) makes it impossible for certain pathogens to infect and reproduce in those cells.
Acquired immunity is just that: resistance to a pathogen that has been acquired or developed. Involving the production of antibodies, that resistance may evolve actively or passively.
Active immunity is formed when B cells encounter antigens and produce antibodies against them. As an individual grows from birth and develops bacterial and viral infections, antibodies are produced in the body to defend it against subsequent attacks. During a lifetime, an individual's active immunity is continually updated and enlarged. Active immunity may also be gained through artificial means: vaccines. A vaccine is a substance made of weakened or killed bacteria or viruses. When injected (or taken orally) into the body, it stimulates the production of antibodies specific to that particular infectious disease. Too weak to take over the body, the harmful microorganisms are destroyed. In the process, the body has developed memory B cells that can react quickly and effectively when threatened by that particular disease in the future. Developing immunity through deliberate exposure to a disease is called immunization.
Passive immunity differs from active immunity in two ways: the antibodies involved come from an outside source and they provide only short-term resistance. Memory B cells are not produced as a result. An example of passive immunity occurs between a pregnant woman and her fetus. During pregnancy, antibodies produced by the mother cross the placenta (the membrane lining the uterus through which nutrients and oxygen pass from mother to fetus) and enter the fetus's circulation. These antibodies remain for several months after birth, protecting the baby from diseases to which the mother is immune (they remain longer if the mother breast-feeds). They are then naturally removed from the baby's circulation and are not replaced. Antibodies may also be given to individuals who are already fighting infection. Antivenins used to treat poisonous snake or spider bites are examples of such antibody preparations that provide immediate but short-term protection.
Comment about this article, ask questions, or add new information about this topic: