The Cardiovascular System - Workings: how the cardiovascular system functions

In its continuous work, an average heart contracts more than 100,000 times a day to force blood through the thousands of miles of blood vessels to nourish each of the trillions of cells in the body. With each contraction, the heart forces about 2.5 ounces (74 milliliters) of blood into the bloodstream. At an average adult heart rate of 72 beats per minute, this equals about 1.4 gallons (5.3 liters) of blood every minute, 84 gallons (318 liters) every hour, and 2,016 gallons (7,631 liters) every day. With exercise, this amount may be increased by as much as five times.
Cardiac cycle
Cardiac cycle refers to the series of events that occur in the heart during one complete heartbeat. Each cardiac cycle takes about 0.8 second. During this brief moment, blood enters the heart, passes from chamber to chamber, then is pumped out to all areas of the body. Each cardiac cycle is divided into two phases. The two atria contract while the two ventricles relax. Then, the two ventricles contract while the two atria relax. The contraction phase, especially of the ventricles, is known as systole; the relaxation phase is known as diastole. The cardiac cycle consists of a systole and diastole of both the atria and ventricles.
In order to increase their endurance before competition, some athletes resort to a technique known as blood doping. The procedure involves withdrawing some of the athlete's red blood cells. After the blood is removed, the athlete's body responds by quickly producing more red blood cells to replace those withdrawn. Then, a few days before a competitive event, the withdrawn blood is infused back into the body.
The effect is to create a greater number of red blood cells and, in turn, a greater concentration of oxygen in the blood. Blood doping can increase an athlete's aerobic capacity by up to 10 percent.
However, blood doping is not only illegal but risky. It can impair blood flow as well as cause flulike symptoms. Instead of helping an athlete's performance, it can hinder it.
The process begins as deoxygenated (carrying very little oxygen) blood returns to the right atrium of the heart via the venae cavae. At the same time, oxygenated blood transported from the lungs by the four pulmonary veins empties into the left atrium. The AV valves open, and as blood flows into the atria it also flows passively into the ventricles. The semilunar valves, however, are closed to prevent blood from flowing out of the ventricles into the arteries. When the ventricles are about 70 percent full, the SA node sends out an impulse that spreads through the atria to the AV node. The atria contract, pumping out the remaining 30 percent of blood into the ventricles.
The AV node slows the impulse briefly, allowing the atria time to complete their contraction. The impulse then travels through the AV bundle, the bundle branches, and the Purkinje fibers to the apex of the heart. As the contraction of the ventricles is initiated at this spot, pressure begins building rapidly in the ventricles and the AV valves close (the "lub" sound heard through a stethoscope) to prevent blood from flowing back into the atria. When the pressure in the ventricles becomes higher than the pressure in the large arteries leaving the heart, the semilunar valves are forced open and blood is pumped out of the ventricles. Deoxygenated blood in the right ventricle is pumped to the lungs via the pulmonary arteries; oxygenated blood in the left ventricle is pumped to the rest of the body via the aorta.
While the ventricles are contracting (systole), the atria are at rest (diastole) and are filling with blood once again. When all the blood is pumped from the ventricles, the semilunar valves close (the "dup" sound heard through a stethoscope) to prevent the backflow of blood into the heart. For a moment, the ventricles are empty, closed chambers. When the pressure in the atria increases above that in the ventricles, the AV valves are forced open and blood begins to flow into the ventricles, starting a new cardiac cycle that will take less than one second to complete.
In short, during the cardiac cycle, the upper half of the heart (the atria) receives blood. The lower half (the ventricles) then pumps out the blood. The right side of the heart (right atrium and right ventricle) receives and pumps out deoxygenated blood; the left side (left atrium and left ventricle) receives and pumps out oxygenated blood.
Blood pressure
When the ventricles contract, they force or propel blood from the heart into the large, elastic arteries that expand as the blood is pushed through them. The pressure the blood exerts against the inner walls of the blood vessels is known as blood pressure. This pressure is necessary to keep the blood flowing to all areas of the body and then back to the heart.
Blood pressure is greatest in the large arteries closest to the heart. Because their walls are elastic, the arteries are able to recoil and keep most of the pressure on the blood as it flows away from the heart. As the blood courses through the system in less elastic vessels—arterioles into capillaries into venules into veins—blood pressure drops. When the blood finally returns to the right atrium via the venae cavae, the pressure behind it is almost zero.
Since the heart contracts and relaxes during a cardiac cycle, blood pressure rises and falls during each beat. It is higher during systole (left ventricle contracting) and lower during diastole (left ventricle relaxing).
Blood pressure is measured in millimeters of mercury (mmHg) with a sphygmomanometer (see box). A blood pressure reading is most often taken on the brachial artery in the arm. The systolic pressure is recorded first, followed by the diastolic pressure. Average young adults have a blood pressure reading of about 120 mmHg for systolic pressure and 80 mmHg for diastolic pressure (written as 120/80 and read as "one-twenty over eighty"). Depending on age, sex, weight, and other factors, normal blood pressure can range from 90 to 135 mmHg for the systolic pressure and 60 to 85 mmHg for the diastolic pressure. Blood pressure normally increases with age.
Regulating the heart rate
Under normal circumstances, the heart controls the rate at which it contracts or beats. But another body system—the nervous system—can and does affect heart rate to help the body adapt to different situations.
Medical personnel measure a person's blood pressure using an instrument called a sphygmomanometer. This device consists of a rubber cuff, a hand bulb pump, and a pressure-reading mechanism.
The rubber cuff of the sphygmomanometer is wrapped snugly around a patient's arm just above the elbow. The individual taking the blood pressure then places a stethoscope (a hearing device) against the patient's brachial artery on the inside of the arm just above the elbow to listen for the pulsing of the blood.
The cuff is then inflated using the hand bulb pump until the blood flow into the arm is stopped and a pulse cannot be heard or felt. The pressure in the cuff is then released slowly. When a small amount of blood begins to spurt through the constricted artery, soft tapping sounds are heard through the stethoscope. The cuff pressure reading at which the first sound is heard is recorded as the systolic pressure.
As the pressure in the cuff is released further, the tapping sounds become louder, then soon soften. When the artery is no longer constricted and blood flows freely, the sounds disappear. The cuff pressure reading at the last sound heard is recorded as the diastolic pressure.
The medulla oblongata is a mass of nerve tissue at the top of the spinal cord and at the base of the brain that controls involuntary processes such as breathing and heart rate. Inside the medulla are two cardiac centers, the accelerator center and the inhibitory center. These centers send nerve impulses to the heart to regulate its beating.
The autonomic nervous system is a division of the nervous system that affects internal organs such as the heart, lungs, stomach, and liver. It functions involuntarily, meaning the processes it controls occur without conscious effort on the part of an individual. The autonomic nervous system is divided into two parts, the parasympathetic and sympathetic systems. The parasympathetic system is active primarily in normal, restful situations; the sympathetic system is most active during times of stress or when the body needs energy.
The accelerator center in the medulla sends impulses along sympathetic nerves to the heart to increase heart rate and the force of contraction. The inhibitory center sends impulses along parasympathetic nerves to the heart to decrease heart rate. The centers act in response to changes in blood pressure and the level of oxygen in the blood, often brought about by factors such as exercise, increased body temperature, and emotional stress. Such changes are detected by receptors located in the carotid arteries and the aortic arch.
Receptors in the carotid arteries detect a decrease in blood pressure; those in the aortic arch detect a decrease in the level of oxygen in the blood. Both send out impulses along sensory nerves to the accelerator center, which in turn sends impulses along nerves to the SA node of the heart to increase heart rate. When blood pressure or blood oxygen level has been restored to normal, the inhibitory center sends out impulses along nerves to the SA node to slow heart rate to a normal resting pace.
Exchanges between capillaries and general body tissues
Arteries, arterioles, venules, and veins: the only function of these vessels is to transport blood from or to the heart. The exchange of materials—oxygen, carbon dioxide, nutrients, and wastes—between the blood and interstitial fluid occurs through the capillaries. The movement of these materials is variously brought about by three processes: diffusion, filtration, and osmosis.
Diffusion is the movement of molecules from an area of greater concentration (existing in greater numbers) to an area of lesser concentration (existing in lesser numbers). Diffusion takes place because molecules have free energy, meaning they are always in motion. This is the case especially with molecules in a gas, which move quicker than those in a solid or liquid. Oxygen and carbon dioxide, the gases that pass between the capillaries and the interstitial fluid, move by diffusion. As blood courses through a capillary, the oxygen carried by the hemoglobin in red blood cells exists in a greater amount and thus moves into the surrounding interstitial fluid to be taken up by the cells. Conversely, carbon dioxide exists in a greater amount in the interstitial fluid and so moves into the capillary to be carried away. This exchange of gases between the blood and the interstitial fluid is called internal respiration.
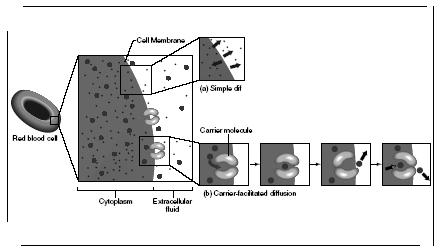
Filtration is the movement of water and dissolved materials through a membrane from an area of higher pressure to an area of lower pressure. When blood enters capillaries, it has a pressure reading of about 33 mmHg; the pressure of the interstitial fluid is only about 2 mmHg. Thus, through filtration, plasma and nutrients such as amino acids, glucose, and vitamins are forced through the capillary walls into the surrounding interstitial fluid.
Osmosis is the diffusion of water through a semipermeable membrane (a membrane that allows some materials but not others to flow through it). It is the movement of water from an area where it is abundant to an area where it is scarce or less abundant. Directly related to this is osmotic pressure, which is the tendency of a solution to "pull" water into it. The strength of this pressure is determined by the amount of dissolved material, called solutes, in the solution. The greater the amount of solutes, the lower the amount of water in that solution. A solution containing a high amount of solutes has a high osmotic pressure, and water has a greater tendency to move into the solution.
At the venous end of capillaries, just before they merge to form venules, the osmotic pressure is greater in the capillaries than in the interstitial fluid. This is due to the presence of albumin and other large proteins that have
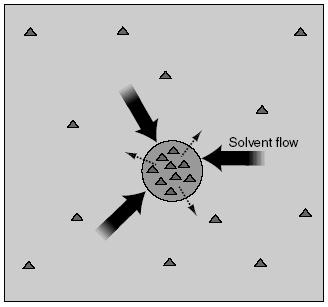
remained as solutes in the blood. Interstitial fluid has a low osmotic pressure and is thus "pulled" into the capillaries and carried away.
Capillary exchange in the lungs
After blood has flowed through the tissues of the body, exchanging oxygen and nutrients for carbon dioxide and wastes, it heads back to the heart. The deoxygenated blood empties into the right atrium via the venae cavae, then into the right ventricle. From here it is pumped into the pulmonary trunk, which then divides into the right and left pulmonary arteries. These arteries transport the deoxygenated blood to each lung.
In the lungs, the arteries branch out into successively smaller arteries and successively smaller arterioles. Finally, the smallest arterioles branch into capillaries. These pulmonary capillaries surround the alveoli, the air sacs of the lungs. The exchange of oxygen and carbon dioxide in the lungs, known as external respiration, takes place across the walls of the alveoli and nearby capillaries.
As in internal respiration, the exchange of gases in external respiration occurs according to the process of diffusion. Air in the alveoli has a high concentration of oxygen. The blood in the pulmonary capillaries has a high concentration of carbon dioxide. Following diffusion, oxygen in the alveoli moves into the capillaries while carbon dioxide in the capillaries moves into the alveoli.
Now oxygenated, blood flows from the capillaries into venules, which merge to form larger and larger veins. Finally, the blood exits each lung through two large pulmonary veins and is carried to the left atrium to be pumped back into the systemic circulation once again. The movement of blood from the lungs to the heart is a special occurrence in the body: it is the only time that veins carry oxygenated blood.
Hepatic portal circulation
Another unique circulation route is the hepatic portal circulation, a subdivision of the systemic circulation. Under this circulation pathway, blood from the digestive organs and the spleen flow through the liver before heading to the heart.
Capillaries that drain the stomach, small intestine, colon, pancreas, and spleen flow into two large veins, the superior mesenteric and the splenic. These two veins then unite to form the portal vein, which carries the blood into the liver.
Once in the liver, the portal vein branches to form capillaries called sinusoids. Sinusoids are larger than normal capillaries. Their walls are also more permeable, allowing proteins and blood cells to enter or leave easily. This is important since the blood entering the liver from the digestive organs contains large amounts of nutrients.
As the blood flows slowly through the sinusoids in the liver, some of these nutrients are removed from the blood and either stored in the liver for later use or changed into other materials the body needs. From the sinusoids, blood flows into the right and left hepatic veins, then into the inferior vena cava, and finally into the right atrium.
The complete flow of blood from the digestive organs to the heart is unusual. Normally, arteries flow into capillaries, which flow into veins. In the hepatic portal circulation, no arteries are involved. Here, capillaries merge to form veins, which branch into capillaries that merge again to form veins. This strange route is necessary so that blood may be altered by the liver. Nutrients may be stored or changed and possible poisons (such as alcohol and medicines) may be transformed into less harmful substances before the blood returns to the heart and the rest of circulation.
Comment about this article, ask questions, or add new information about this topic: